Nokia fixes mmWave wireless access
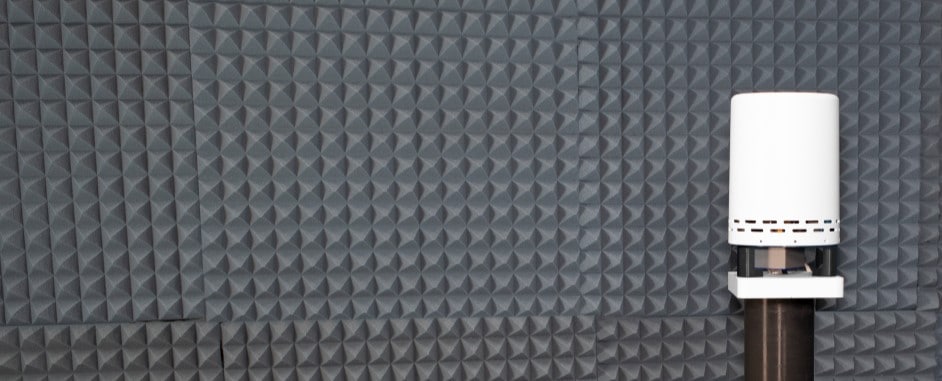
We’ve written a few times about the vital role fixed wireless access (FWA) plays in connecting the unconnected. Yes, we still all believe in a full-fiber future, but the arrival of 5G finally made FWA a fantastic option for broadband where it is currently too complex or costly to draw fiber all the way to premises. With additional 5G spectrum in the mmWave bands becoming available in every major market, FWA can shine even more. The increased bandwidth of this mmWave band enables FWA to deliver gigabit broadband speeds over several kilometers.
But of course, like every new technology, there are some challenges to overcome. mmWave’s increased bandwidth comes at the cost of far greater susceptibility to signal loss. Firstly, losing line-of-sight cuts down the coverage area tremendously, seriously limiting real-life deployment scenarios. Secondly, avoiding this loss of line of sight means engineers are needed for every installation, adding a significant financial barrier to adoption.
These are exactly the kinds of challenges we relish at Nokia. And I’m proud to reveal that we have found solutions to them.
We have put some technology innovations into a 5G FWA CPE, tested them in our lab, tested them on our campus in Espoo, Finland, and trialed them in real-life high-density urban environments. And the results, which I shall come onto, are a resounding success.
But first; the technology breakthroughs.
To overcome the need for consistent line-of-sight, we have developed a very high-gain antenna with a 360° field of view. The high gain means our CPE can take advantage of weaker reflected or refracted signals even when line-of-sight is lost. To get the highest benefit, we need to communicate over the strongest possible signal. To automatically find that optimal signal, we created a few intelligent algorithms. Firstly, we scan the indoor environment to create a fingerprint of where signals are coming from. Then, if signal strength drops below a certain threshold - say, when a pesky human walks in front of the window - the CPE instantly switches to the best alternative signal. We even made the CPE dual-band so that, in the event that no mmWave signal is strong enough, it can still guarantee a minimum level of service using sub-6 GHz spectrum in all conditions.
Figure 1: mmWave signal loss and self-install challenges
Pretty neat, right? But the most promising aspect of this technology is that in this setup the precise location and orientation of the mmWave CPE is no longer a critical success factor, allowing for customer self-install deployments which greatly reduce costs and accelerate time-to-service.
Through our work we figured out an ideal deployment scenario for mmWave FWA, as an offload for sub-6 GHz traffic when the network gets too busy. Our dual-band model is simpler to achieve than densification so can be used the majority of the time, reserving a more costly densification approach for delivering high sustained rates in situations where the take-rates or market price make it commercially attractive. Of course, dedicated mmWave deployments for dense urban environments are still an option, and we will also continue to advocate for long-reach deployments of mmWave when line-of-sight can be guaranteed, as that’s a fantastic way to reach very remote communities and homesteads.
It’s more important than ever that we accelerate connectivity to those who are socially and economically vulnerable because they do not have a broadband connection good enough for the new normal. We believe our mmWave FWA technology innovations pave the way for products that can do exactly that, benefitting customers, operators, and society as a whole in the not-too-distant future.
To learn more, download our exclusive technology white paper.