How to build better IP services with segment routing
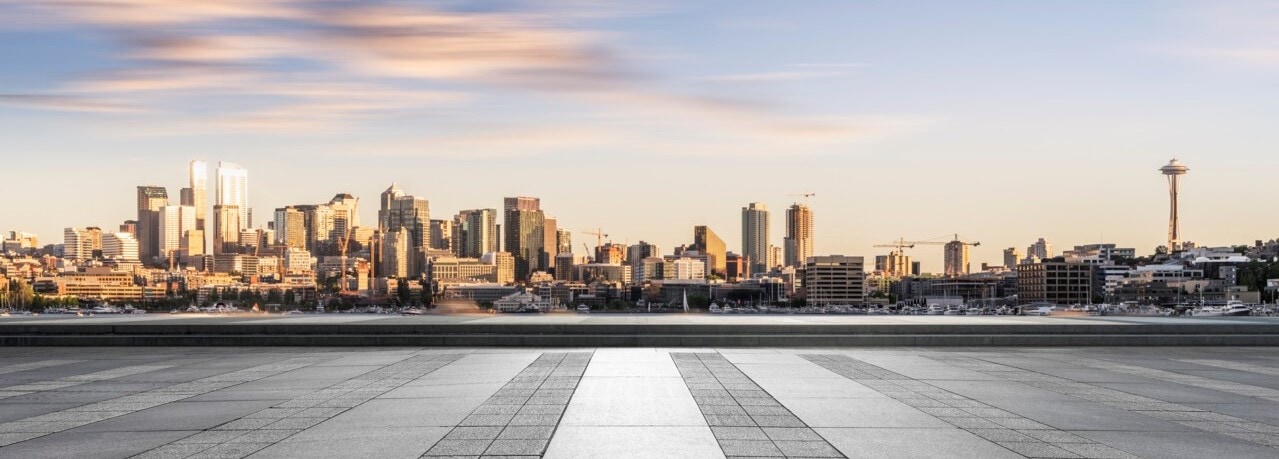
Growing up in the Netherlands, I enjoyed watching a popular TV quiz show that featured a hamster maze. Contestants would pick a hamster and let it run through a maze until it reached a set of portals marked with different prize nominations. To increase the odds, they could tinker with the maze and strategically place their hamster at the entrance. It was great fun watching the creature navigate its way through the maze and hearing the cheers and jeers of the audience as it dashed through a portal to determine how much money each candidate had won.
IP packets are generally more successful than hamsters in ending up at the desired destination port, but network operators often don’t have much control over how they get there either. For IP service traffic, the journey is often as important as the destination, and tinkering with IP link metrics does little to help guide the flow of packets through the network. More effective tools are needed to reliably control and steer IP traffic through the network along optimal routes.
Why your network needs segment routing
Segment routing (SR) offers a scalable approach for establishing predefined forwarding paths in the IP network that override the default shortest path while meeting specific constraints like available bandwidth, latency and physical diversity. SR removes the need for resource-intensive, per-path control plane signaling and keeps a minimum of forwarding state information on routing nodes. As a result, it scales better than conventional approaches based on RSVP-traffic engineering (RSVP-TE) or label distribution protocol (LDP) and can engineer more granular traffic flows.
Segment routing is supported by a rich toolkit that consists of various functional layers with several options for implementing each layer depending on the service requirements (Table 1).
Table 1. Segment routing toolkit
Segment routing takes a source-based routing approach, which requires only the ingress or head-end router to maintain policy and state information about the path. In its simplest form, a segment route is a sequence of segments that act as waypoints for forwarding packets along a constrained path that meets a given policy. Segments can refer to different network objects such as subnets, routing nodes or interfaces to be included or excluded in the data path.
Segment routing for MPLS (SR-MPLS) supports both IPv4 and IPv6 data planes while SRv6 is dedicated to IPv6. Traffic engineering is supported through SR-TE label switched paths (LSPs) or SR policies and both options provide a logical traffic tunnel that adheres to specific forwarding constraints. From a user perspective, SR-TE LSPs are similar to traditional, traffic-engineered LSPs such as RSVP-TE and offer a natural migration path. Segment routes can be engineered and programmed by a centralized software defined networking (SDN) controller or determined by edge routers themselves based on policy constraints.
Segment routing can also be applied to pre-calculate backup paths that protect against failure of the primary path. Primary and secondary paths can be defined with strict or loose hops and initiated by a router or by an external path computation element (PCE) controller. Fast reroute is supported through the ability to precompute alternate next-hop backup paths called loop-free alternates (LFAs), to quickly take over if a failure is detected.
How to introduce segment routing to your network
Because SR-MPLS supports native IPv4 and IPv6 data planes without adding packet processing requirements, it can be deployed on existing hardware and yield immediate service benefits, without sacrificing any transport capabilities offered by LDP and RSVP-TE. Using the segment routing toolkit, operators can incrementally add the features they need to an existing LDP/RSVP network with the options they are comfortable with. This approach eases migration and allows the operator to gain operational experience before introducing more powerful features (see Figure 1).
Figure 1. Segment routing applications
Shortest path routing is a good starting point for introducing SR as a replacement for LDP and requires control plane extensions only for IS-IS or OSPF.
Constraint-based shortest path routing could be a logical next step to engineer low-latency paths for delay-sensitive service applications or to ensure traffic flows are kept within a controlled set of links.
Path diversity and end-to-end protection enable highly available, premium services as a more scalable alternative for MPLS fast reroute based on RSVP-TE.
More advanced SR-TE applications such as peering engineering, transport slicing, service chaining and flow steering are enabled by additional programmatic capabilities.
Conclusion
Segment routing is a powerful and proven technology for deploying scalable and programmable IP services that meet deterministic service level objectives for cost, performance and reliability. Segment routing addresses the operational scalability issues of legacy traffic engineering and protection approaches and enables a wide range of new applications, especially in combination with the Nokia Network Services Platform (NSP).
The Nokia Service Router OS (SR OS) offers a comprehensive segment routing toolkit for IP/MPLS networks, next-generation IPv6 networks and the transition between them. To learn more about how your network will benefit from these capabilities, read this application note.