How innovations in spectrum use enable continued growth in optical fiber capacity
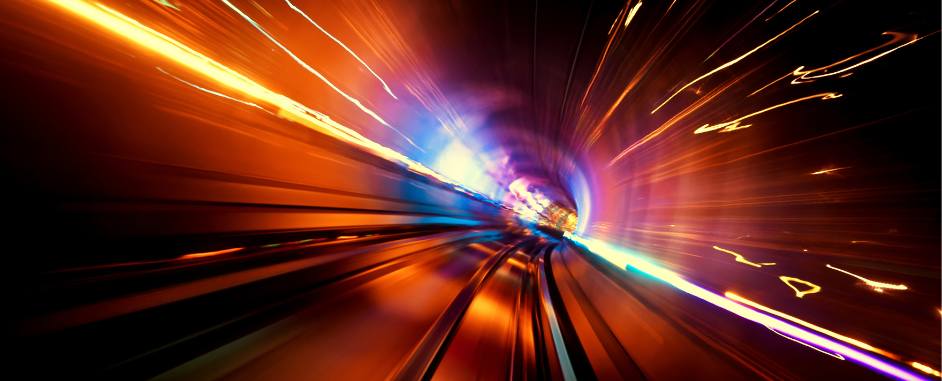
In my last blog, I started to answer the question: how we are going to scale optical transmission to meet the ever-growing demand, which is currently at about 40 percent per year? I looked at the elements of the Shannon Limit and what we have achieved in spectral efficiency, where we are now operating close to that limit. In this blog, I will look at “B”, which in Shannon’s equation signifies the amount of bandwidth. To scale bandwidth, light can be transmitted through the core of optical fibers over a wide range of frequencies, also known as wavelengths or colors. In fact, the useable spectrum of optical fiber for optical communications is much broader than the range of the visible spectrum that humans can detect.
For optical communications, spectrum is defined by the operating range of the optical fiber over which wavelength signals, each carrying a data stream, can be propagated over long distances. Just like lanes on a highway, as more spectrum is available for transmitting different wavelengths, more data channels can be transmitted in parallel. The total amount of usable spectrum available is defined by the characteristics of the fiber. Thus, the more spectrum of the optical fiber can be used, the greater the metric “B” or Bandwidth in the Shannon equation.
Spectrum scaling
Before we explore how to utilize more fiber spectrum, let’s take a step back and review a similar technology scaling paradigm in an adjacent field; namely that of silicon-based microprocessors over the past 50 years. This can provide a perspective on how these learnings can be applied to tackle future scaling in optical fiber communications while considering the attributes that are unique to optical transmission systems.
From their inception, the single-thread core performance of central processing units (CPUs) in microprocessors doubled for many years, thanks to the reduction in size and doubling of transistors being packaged on a silicon die; famously coined as Moore’s Law. This was accomplished through continued reductions in transistor size, leading to decades of bi-yearly doubling of processing performance. This parallels how ongoing increases in spectral efficiency, as described in my first blog, were enabled by continued increases in the transmission rate of coherent optics.
Microprocessor vendors originally expected that by packaging more transistors into a smaller area using new process nodes, circuits could operate faster and with lower power consumption. Unfortunately, this was not the case. Smaller transistor devices had thinner dielectrics and shorter channels were more prone to current leakage, which caused junction temperatures to rise significantly, thus thermally limiting switch clock frequencies to no greater than 4GHz.
This created a barrier to further scaling that couldn’t be addressed simply by further reductions in transistor size. To continue scaling in line with Moore’s Law, microprocessor vendors such as Intel and AMD introduced the industry’s first multi-core CPUs circa 2005, which enabled lower power consumption and mitigated the need for further increases in clock speeds.
As depicted in Figure 1a, the move to multicore architectures and parallel processing enabled the continued increases in the processing power of CPUs for another decade or more, until recently when new technology challenges are again complicating a path to further scale.
![]() |
![]() |
Figure 1a. Fifty years of microprocessor data trends | Figure 1b. Continued evolution in optical fiber capacity enabled by WDM |
It is interesting to observe how microprocessor capacity evolution is analogous to approaches that have been developed to scale optical fiber capacity. As with the innovation of multicore architectures in microprocessors, wavelength division multiplexing (WDM) used parallelism, combining many data transmitting optical channels, each operating on different wavelengths transmitted over a single optical fiber, to help scale fiber capacity over one hundredfold in the past 25 years, as shown in Figure 1b.
Do WDM techniques still have room to scale? The number of channels available for WDM transmission depends on how much spectrum is available. We will focus on what techniques have been utilized to maximize the spectrum available today and explore further innovations that could help push these limits even further.
Let’s start with optical spectrum. The optical fiber plays a key role in defining the total capacity that can be transmitted. This usable spectrum of optical fiber utilizes multiple bands, termed O, E, S, C, L and U, as shown in Figure 2 along with their fiber loss characteristics. The fiber loss is important, as it degrades the intensity of optical signals and limits the signal-to-noise ratio (SNR) of a data channel and, thus, it’s reach. These characteristics determine which parts of the optical fiber spectrum can feasibly be considered for use.
Figure 2. Operating spectrum of optical fibers, and different spectrum bands available for WDM
The spectrum traditionally used for optical transmission at distances over 10km has been in the bands having the lowest loss propagation through the fiber. This has led to widespread use of the C-Band, from 1530-1565 nm, which has both low propagation loss and aligns well with the operating range of Erbium-doped fiber amplifiers (EDFA). These can amplify multiple wavelengths simultaneously, making dense wave-division multiplexing (DWDM) possible over long distances, and economical by avoiding the need to amplify every channel individually. In fact, the available bandwidth for optical communication has been limited by the bandwidth of the EDFAs used for long distance communication.
Further scaling of the optical transport capacity beyond the C-band led to the use of the L-band. The L-band, adjacent to the C-band at a lower frequency, also exhibits low propagation loss, however, it required innovations to expand the operating range of optical technologies such as lasers, modulators and detectors, amplifiers, and ROADMs.
Nokia introduced the first widely deployed coherent DWDM optical transmission systems operating in the L-band and opened a whole new transmission spectrum from 1565nm to 1625nm. This effectively doubled the number of wavelengths that can be used for transmission, and enabled network operators to expand their C-band networks to C + L band, over existing deployed fiber. These L-band capable solutions include Reconfigurable Optical Add/Drop Multiplexers (ROADMs), L-Band line amplifiers, and coherent transponders able to tune across the C-Band and L-Band.
Initially, network operators deploying C + L band networks were those that had high traffic demands and fiber-constrained long-haul networks. These included hyperscalers, who took advantage of C + L technology to build “super-highways” across continents. Other operators, such as those in Japan or Italy, deployed L-Band solutions to maximize WDM capacity over networks deployed with Dispersion Shifted Fiber (DSF), which could not scale in the C-band due to the nonlinear distortions called four-wave mixing.
Today, C + L band solutions are being deployed broadly across the globe in both metro and long-haul networks by a wide range of network operators including CSPs, cable operators and enterprise customers. The availability of C + L band solutions is enabling network operators to double their network capacity and avoid exhausting the fiber capacity of their installed optical fiber cables. These solutions helped reduce or defer large capital investments for fiber cable over-builds, and made possible new business models for wholesaling the increased spectral capacity. Our innovation in this area has led to Nokia becoming a market leader in the deployment of L-band solutions.
The question now is whether it is possible for WDM optical transmission to scale, beyond C and L bands? The short answer is “Yes, it is possible,” however, there is much work to be done.
Recent technology developments are presenting an opportunity to expand into the S-Band from the adjoining C-band, making it attractive to further extend WDM operation into the spectrum from 1460nm to 1530nm. One approach relies on the use of semiconductor optical amplifiers (SOAs) to cover the S-Band spectrum. An advantage of SOAs is that their amplification gain profile can be tailored through suitable III-V material design and semiconductor bandgap engineering so that they can operate across a wide range of wavelengths used for optical communications. SOAs have also been demonstrated to have a large enough output power to rival EDFA technology, with a compelling noise figure that is in reach of industrialization.
Recently, Nokia Bell Labs researchers published in the Journal of Lightwave Technology (Vol 38:5, Mar 2020, pp 1071-1079) a successful demonstration of the transmission of 115 Tb/s of data across 100nm of spectrum using an ultra-wide band SOA operating from 1510–1610nm. By increasing available spectrum from the C-band to also include the L-band and the S-band, network operators will be able to access up to ~60nm of usable spectrum, and further improve total fiber capacity.
Furthermore, and very importantly, SOAs can be monolithically integrated with lasers, modulators and photodetectors into multi-function photonic integrated circuits (PIC), enabling further advances in device integration and resulting benefits in size, performance and lower power. These recent developments highlight the progress being made in developing new technologies that can further scale WDM capacity in the future.
The dawn of a new era
Fiber utilization refers to the use of the available spatial capacity of the network, either the number of fibers used, the number of cores in the fiber through which light is transmitted, or both. Single mode fiber used for optical fiber transmission networks, can be manufactured so that all the data travels along a single core, at its center, or in the case of multi-core fibers, across multiple cores within 125micrometers.
In the final blog in this series, we will uncover what is new and available in optical fiber types and provide a glimpse of a new area of research called spatial propagation that promises further transmission capacity gains.
Stay tuned!